渗流泡沫
铝独特的开孔结构,使其具有低密度、高比强度、耐温、不吸湿和可回收等特性[1~5]
这种泡沫铝对频率高于800Hz的噪声有良好的吸收性能[6],有广阔的应用前景[7~12]
渗流泡沫铝的孔隙率,对其吸声性能有显著的影响[13~19]
低孔隙率(孔隙率低于70%)渗流泡沫铝在1000~5800 Hz的平均吸声系数低于0.7[6]
随着孔隙率的提高其吸声性能提高,但是力学性能严重降低[20, 21]
Wang Hui等[22]发现,孔隙率从61.5%提高到65.5%时,泡沫铝的吸声系数增大了11.24%;Liu[23]制备的孔隙率为85%~96%的高孔隙率渗流泡沫铝,其屈服强度只有0.13~0.28 MPa;Wu[21]采用熔模铸造法制备的孔隙率为85%~93.5%的开孔泡沫铝,其屈服强度也只有0.086~0.102 MPa
改性处理和T6热处理使泡沫铝的屈服强度有所提高(0.235 MPa),但是仍然不能满足作为结构材料使用的要求
制备兼具高力学性能及高吸声性能的渗流泡沫铝,是重要的研究方向
提高渗流泡沫铝力学性能最有效方法,是对泡沫基体进行复合化处理,用颗粒、晶须或纤维等材料使其强化 [24~31]
Altink?k[24]研究了Al2O3/SiCp
复合材料,发现起抗拉强度随着SiCp粒径的减小而提高;郭成等[25]制备了MgAl2O4晶须增强铝基复合泡沫材料,发现MgAl2O4晶须使其压缩性能大大提高;Zhang等[27]制备了编织
碳纤维增强铝基复合材料,发现纤维复合使其力学性能明显提升;Mu等[28]制备了碳纤维/泡沫铝复合材料,发现纤维含量(体积分数)为5%的复合泡沫其压缩性能比纤维含量为1%的复合泡沫明显提高
本文制备ZL104合金泡沫和304不锈钢纤维/ZL104合金复合泡沫,研究孔隙率和纤维含量对复合泡沫力学性能和吸声性能的影响并分析其机理
1 实验方法1.1 试样的制备
基体材料为ZL104
铝合金(9.3%Si,0.25%Mg和0.32%Mn),渗流前驱体为NaCl颗粒,其直径分别为0.35 mm(主盐)和0.12 mm(调控盐),比例为7∶1.2,增强体为304不锈钢纤维(?0.1 mm×5 mm)
将前驱体颗粒和不锈钢纤维均匀混合后压制成预制体,然后将其加热到650℃,保温30 min后用ZL104合金熔体进行压力渗流(渗流压力0.4~0.8 MPa)
渗流体凝固后用水将其中的盐颗粒溶除,通过工艺调控制备的试样参数列于表1
试样的孔隙率范围为77%~86%,制备的合金泡沫(不含纤维的泡沫,1#~5#)及复合泡沫(6#~13#)纤维含量(体积分数)分别为0%、2%、5%、8%和11%
Table 1
表1
表1合金泡沫和复合泡沫试样的参数
Table 1Sample parameters of alloy foams and composite foams
Sample | Pore size/mm | Porosity/% | Fiber content/volume fraction, % | Fiber size/mm2 |
---|
1# | 0.35+0.12 | 77 | 0 | … |
2# | 0.35+0.12 | 80 | 0 | … |
3# | 0.35+0.12 | 82 | 0 | … |
4# | 0.35+0.12 | 84 | 0 | … |
5# | 0.35+0.12 | 86 | 0 | … |
6# | 0.35+0.12 | 77 | 8 | ?0.1×5 |
7# | 0.35+0.12 | 80 | 8 | ?0.1×5 |
8# | 0.35+0.12 | 82 | 8 | ?0.1×5 |
9# | 0.35+0.12 | 84 | 8 | ?0.1×5 |
10# | 0.35+0.12 | 86 | 8 | ?0.1×5 |
11# | 0.35+0.12 | 82 | 2 | ?0.1×5 |
12# | 0.35+0.12 | 82 | 5 | ?0.1×5 |
13# | 0.35+0.12 | 82 | 11 | ?0.1×5 |
1.2 性能表征
(1) 用SEM(S-3400N型)表征孔结构,包括孔形貌、纤维分布以及纤维-基体结合情况;用盐颗粒粒径、结合扫描电镜表征孔径;用纤维与复合泡沫基体的体积比表征纤维含量;孔隙率为
?=1-ρ1ρ0?100%=(1-MV?1ρ0)?100%(1)
式中?为泡沫孔隙率;ρ0为ZL104铝合金密度(2.65 g/cm3);ρ1为泡沫试样密度,用排水法测量;M为泡沫试样质量;V为泡沫试样体积
(2) 用Autograph AG-X100KN万能试验机测试泡沫试样(直径和厚度为30 mm)的压缩性能,对试样准静态压缩的速率为3 mm/min
(3) 根据国家标准GB/T 18696.2-2002,基于传递阻抗法,在刚性背衬条件下用SW-477阻抗管测试泡沫试样(直径和厚度为30 mm)在1000~5800 Hz频率范围内的垂直入射吸声系数
(4) 有限元模拟:使用COMSOL Multiphysics ?有限元软件对复合泡沫压缩过程进行有限元模拟,结合复合泡沫的力学性能分析纤维复合对复合泡沫力学性能的影响机理
(5) J-A模型分析:采用J-A模型表征复合泡沫的吸声性能,结合测量的复合泡沫的吸声系数分析纤维影响复合泡沫吸声性能的机理
2 结果和讨论2.1 ZL104合金泡沫和304不锈钢纤维/ZL104合金复合泡沫的孔结构
图1给出了ZL104合金泡沫和304不锈钢纤维/ZL104合金复合泡沫的孔结构
由图1a可见,合金泡沫的孔结构均匀,孔径偏差较小,大部分孔隙呈扁圆形,孔隙间彼此贯通,几乎看不到闭孔结构;由图1b、c可见,纤维复合后孔壁的粗糙度和孔结构的复杂程度明显提高,部分孔壁变为纤维/合金复合孔壁,孔壁也比合金泡沫的更薄;另外,在合金泡沫和复合泡沫孔壁上都有次孔结构(图1a、b)
其原因是,在预制体混合和压制过程中粒径为0.12 mm的调控盐颗粒填充在粒径为0.35 mm主盐颗粒间隙中,渗流后去除预制体形成了多孔孔壁结构
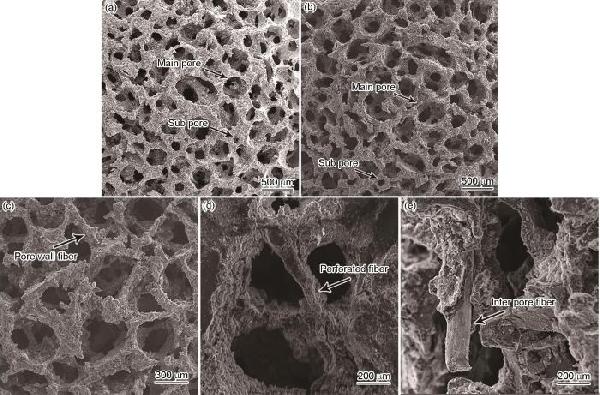
图1ZL104合金泡沫和304不锈钢纤维/ZL104合金复合泡沫的孔结构以及复合泡沫中纤维的状态
Fig.1Pore structure of ZL104 alloy foam (a) and 304 stainless steel fiber/ZL104 alloy composite foam (b) and fiber in the composite foam (c~f)
由图1c、d、e可见,纤维不规则地分布在孔壁和孔隙与孔隙之间的合金基体上,有(i)孔壁纤维、(ii)穿孔纤维和(iii)孔间纤维(部分嵌入孔壁、端头部分延伸到孔隙中)三种形态
嵌入孔壁中的纤维(i)和(ii)有利于复合泡沫保持较高的强度,而声波与孔壁和孔壁上的纤维(ii)和(iii)产生的摩擦增大可提高吸声性能
2.2 力学性能2.2.1 孔隙率对合金泡沫和复合泡沫压缩性能的影响
图2给出了孔隙率为77%~86%的合金泡沫(图2a,1#~5#)和纤维含量为8%(体积分数)的复合泡沫的应力-应变曲线(图2b,6#~10#),表2列出了不同孔隙率泡沫试样的屈服强度
由图2可见,合金泡沫和复合泡沫的压缩曲线都有金属泡沫典型的压缩三阶段特征:线弹性阶段、塑性应变阶段以及致密化阶段
可以发现,复合泡沫的塑性变形阶段的曲线比合金泡沫的平滑,表明复合泡沫具有比合金泡沫更高的压缩稳定性
另外还可见,在相同的应变条件下复合泡沫致密化阶段的应力值远比合金泡沫的大,可归结于纤维的增强作用
从表2可见,孔隙率由77%提高到86%,合金泡沫相应的屈服强度从1.9 MPa下降到0.5 MPa,复合泡沫相应的屈服强度从2.15 MPa下降到0.7 MPa,合金泡沫和复合泡沫的屈服强度都随着孔隙率的增大而降低,而复合泡沫的屈服强度高于相同孔隙率的合金泡沫
还可以看出,在孔隙率相同的条件下复合泡沫的屈服强度均高于合金泡沫,表明复合泡沫能承受更大的变形量而不至于压塌

图2孔隙率不同的合金泡沫和复合泡沫试样的压缩应力-应变曲线
Fig.2Stress-strain curves of alloy foam (a) and composite foams (b) with different porosity

图3纤维含量不同的复合泡沫的应力-应变曲线
Fig.3Stress-strain curves of composite foams with different fiber contents

图4合金泡沫和复合泡沫试样的吸声系数与频率的关系
Fig.4Relationship between sound absorption coeffic-ient and frequency of composite foams with diff-erent porosity (a) composite foam; (b) Compar-ison of 82% porosity alloy foam and composite foam

图5纤维含量不同的复合泡沫的吸声系数与频率的关系
Fig.5Relationship between sound absorption coefficient and frequency of composite foam with different fiber contents

图6合金泡沫和复合泡沫的压缩应变
Fig.6Compressive strain of alloy foam (a) and composite foam (b)
Table 2
表2
表2孔隙率不同的泡沫试样的屈服强度
Table 2Yield strength of composite foam with different porosity
Porosity/% | 77 | 80 | 82 | 84 | 86 |
---|
Alloy foam/MPa(1#~5#) | 1.90 | 1.30 | 0.80 | 0.70 | 0.50 |
Composite foam/MPa(6#~10#) | 2.15 | 1.50 | 1.63 | 1.10 | 0.70 |
2.2.2 纤维含量对复合泡沫压缩性能的影响
图3给出了孔隙率为82%、纤维含量不同的复合泡沫试样(8#、11#~13#)的应力-应变曲线,表3列出了相应的屈服强度数据
由图3和表3可见,复合泡沫的屈服强度随着纤维含量的提高先提高后降低,最大屈服强度对应的纤维含量即为最佳纤维添加量
纤维含量小于5%的复合泡沫其屈服强度随着纤维含量的提高而提高;纤维含量为5%的复合泡沫(11#)屈服强度最高(2.6 MPa),比合金泡沫(3#)提高了225%;纤维含量高于5%时屈服强度随着纤维含量的继续提高而逐渐降低,强化效果减弱,导致复合泡沫抵抗外力变形破坏能力降低、屈服强度下降,整体仍比合金泡沫的高
Table 3
表3
表3纤维含量不同的复合泡沫的屈服强度
Table 3Yield strength of composite foams with different fiber contents
Sample | 11# | 12# | 8# | 13# |
---|
Fiber content/% | 2 | 5 | 8 | 11 |
Yield strength/MPa | 0.60 | 2.60 | 1.63 | 0.90 |
2.3 吸声性能2.3.1 孔隙率对合金泡沫和复合泡沫吸声系数的影响
图4a给出了纤维含量为8%、孔隙率不同的复合泡沫试样(6#~10#)的吸声系数与频率的关系,图4b比较了孔隙率为82%的合金泡沫试样(3#)和复合泡沫试样(8#)的吸声系数,表4列出了试样的平均吸声系数
由图4a可见,在1000~5800 Hz的声频范围内复合泡沫的吸声系数随频率呈振荡变化,出现吸声峰和吸声谷,并且随着孔隙率的提高吸声峰和吸声谷向高频方向移动
由表4可见,复合泡沫的平均吸声系数随着孔隙率的提高先增大后减小,孔隙率为82%的复合泡沫吸声性能最佳,平均吸声系数为0.893
图4b和表4比较了孔隙率同为82%的复合泡沫与和合金泡沫的吸声性能
与相同孔隙率的合金泡沫(3#)相比,复合泡沫(8#)的吸声峰向低频方向移动并具有更高的平均吸声系数,复合泡沫(8#)的平均吸声系数比合金泡沫(3#)提升了10.2%,说明纤维复合能提高复合泡沫的吸声性能
Table 4
表4
表4孔隙率不同的泡沫试样的平均吸声系数
Table 4Average sound absorption coefficient of composite foam with different porosity
Porosity/% | 77 | 80 | 82 | 84 | 86 |
---|
Alloy foam(1#~5#) | - | - | 0.810 | - | - |
Composite foam(6#~10#) | 0.829 | 0.870 | 0.893 | 0.888 | 0.832 |
2.3.2 纤维含量对复合泡沫吸声系数的影响
图5给出了孔隙率为82%、纤维含量不同的复合泡沫试样(6#~10#)的吸声系数与频率的关系,表5列出了相应的平均吸声系数
由图5和表5可见,复合泡沫的吸声曲线达到峰值频率后,随着纤维含量的提高吸声峰向低频方向移动、峰值增大,吸声性能提高;复合泡沫的平均吸声系数呈先增后减的趋势
纤维含量≤5%时,虽然纤维网络不完整,但是吸声性能比合金泡沫显著提高;纤维含量为8%的复合泡沫(8#)吸声性能最佳,平均吸声系数高达0.893;但是纤维含量高于8%的复合泡沫,吸声性能随着纤维含量的提高逐渐降低
这表明,孔隙率为82%的复合泡沫其最佳吸声性能对应的最优纤维添加量为8%
Table 5
表5
表5纤维含量不同的复合泡沫的平均吸声系数
Table 5Average sound absorption coefficient of composite foam with different fiber contents
Sample | 3# | 11# | 12# | 8# | 13# |
---|
Fiber content/% | 0 | 2 | 5 | 8 | 11 |
Average sound absorption coefficient | 0.810 | 0.882 | 0.884 | 0.893 | 0.872 |
3 机理分析3.1 力学性能的机理
使用COMSOL Multiphysics ?有限元软件,对复合泡沫压缩过程进行有限元模拟,图6给出了合金泡沫和复合泡沫(纤维含量5%)压缩前后的应变分布
图6a给出了合金泡沫的应变分布,图6b给出了复合泡沫的应变分布
在图6a及图6b的椭圆区域,在相同应力条件下对比了孔结构变形前(黑色)、后(蓝色)的形态变化
可以看出,未加纤维区域的应变幅度较大,而有纤维区域的演变幅度较小
其原因是,复合泡沫中的纤维受载荷作用时纤维发生位移和偏转(图6b箭头部分)抵耗了部分压缩能量从而增大了对变形的抗力,使复合泡沫的应变较小
纤维含量为8%的复合泡沫不同位置(A、B、C、D、E、F、G和H)的应力分布,如图7所示
随机选取4个不含纤维和4个含有纤维的孔壁较薄位置进行应力分析,8个位置的应力值列于表6
结合图7和表6可知,复合泡沫受到外力时纤维区域E、F、G、H处的应力值总体上小于无纤维区域的A、B、C、D处
这表明,纤维能将压应力传递和分散,使孔壁的受力均匀化程度提高、降低了应力集中,从而提高了复合泡沫的屈服强度
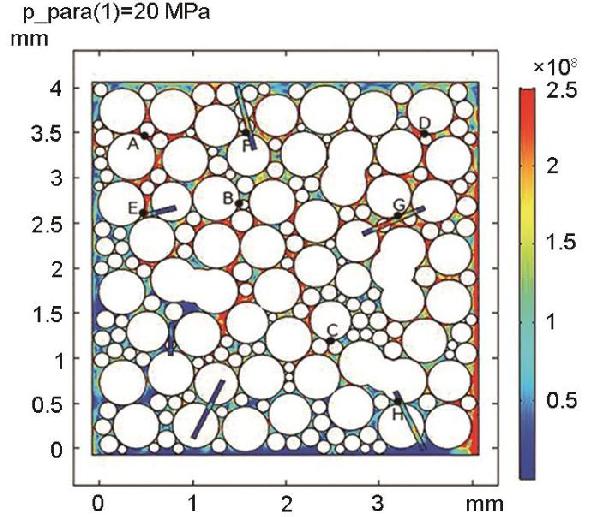
图7纤维含量为8%的复合泡沫不同位置的应力分布
Fig.7Stress of alloy composite foams with fiber content 8% at different positions
Table 6
表6
表6纤维含量为8%的复合泡沫不同位置的应力
Table 6Stress values of composite foam with fiber content of 8% at different locations
Position | A | B | C | D | E | F | G | H |
---|
Stress /×109 Pa | 9.26 | 8.40 | 1.98 | 4.65 | 3.09 | 0.746 | 0.651 | 1.31 |
有限元分析结果表明,在外加载荷相同的条件下复合泡沫的应变和应力均小于合金泡沫,纤维提高复合泡沫力学性能的效果显著;由图1可见,纤维在复合泡沫中的状态主要有孔壁纤维(图1c)、穿孔纤维(图1d)和孔间纤维(图1e)
孔壁纤维和穿孔纤维能在复合泡沫受到外力时产生偏转和位移抵耗部分压缩能量;此外,纤维还能传递和分散应力,降低局部应力集中;纤维还能钉扎裂纹和抑制裂纹扩散、阻碍压缩应变并改变压缩塌陷路径,从而提高复合泡沫抵抗外力变形的能力[28, 32]
随着纤维含量的提高孔壁纤维和穿孔纤维数量、纤维-基体合金结合界面增加,使纤维的增强作用逐渐增大;但是,纤维含量高于5%后,其在泡沫基体中的占比增加使合金基体对纤维的包裹程度降低,降低了纤维增强效果
3.2 吸声性能的机理3.2.1 孔隙率的影响
在孔径相同的条件下,随着孔隙率的提高泡沫孔壁变薄、孔隙数量增多、多孔化程度提高和比表面积增大,入射到泡沫内部的声波与孔壁间的摩擦、反射和衍射程度提高,使声能的衰减加剧
另外,孔壁上的次孔结构还能改变穿过其中的空气流速、扰乱或改变声波的传播,使声波与孔壁摩擦增多从而提高泡沫的吸声性能
但是,由图8可见,孔隙率由82%提高到86%使孔壁的厚度减小并使孔隙合并、孔隙增大和孔壁变薄,导致泡沫的通透性过高和比表面积减小,使声波的传播路径线性化,对声波的摩擦、粘滞和反射等效应减弱,从而使泡沫的吸声性能下降

图8孔隙率不同的ZL104合金泡沫的局部孔结构
Fig.8Local pore structure of ZL104 alloy foam (a) porosity 82% (b) porosity 86%
3.2.2 纤维复合的影响
纤维复合泡沫的吸声性能可用J-A模型[33]描述:
α=1-Z-ρ0c0Z+ρ0c02(2)
Z=-jZc?cotkt(3)
Zc=K(ω)ρ(ω)(4)
k=ωρ(ω)/K(ω)(5)
ρω=ρ0α∞1+σ?jωρ0α∞1+4jα∞2ηωρ0σ2Λ2?21/2(6)
Kω=γP0/γ-γ-11+σ?jB2ωρ0α∞1+4jα∞2ηωρ0σ'2Λ'2?21/2-1(7)
Λ=1c8α∞ησ?1/2(8)
Λ'=1c'8α∞ησ?1/2=8α∞ησ'?1/2(9)
σ=8μ?d2(10)
式中α为吸声系数,Z为泡沫金属表面阻抗,Zc为泡沫金属的特性阻抗,k泡沫金属中的声波传播常数,t为泡沫金属厚度,α∞ 为曲折因子;ρ0为空气密度;c0为空气的声速;σ为静流阻率;?为孔隙率;ω为声波角频率;γ为绝热常数;P0为空气压强;B2 为空气的prandtl数;η为空气粘滞系数;Λ为粘性特征尺度,表征粘滞损耗占主要时孔隙网络的压缩截面的尺度;Λ′为热特征尺度,表征以热损耗为主时孔隙内表面积较大区域的尺度;d为孔径;μ为表征孔壁表面粗糙度的常数
孔径、孔隙率、声频和材料的厚度一定时,复合泡沫的吸声系数主要受孔壁粗糙度μ和曲折因子α∞ 影响
纤维复合后,由于复合泡沫中纤维的含量有限,纤维对曲折度α∞ 的影响较小,因此其影响可以忽略;孔间纤维和穿孔纤维(图1d,e)使复合泡沫孔壁表面的粗糙度μ和泡沫静流阻率σ增大(式10),于是复合泡沫的粘性特征尺度Λ(式8)、热特征尺度Λ'(式9)减小、有效弹性模量Kω(式7)、有效密度ρω(式6)、特性阻抗Zc减小(式4)、表面阻抗Z(式3)减小,吸声系数α(式2)增大
纤维复合后,声波通过孔隙时受到穿孔纤维和孔间纤维以及多孔孔壁的阻碍而发生衍射或绕流,使声波与孔壁之间的碰撞次数增加,还可能带动孔隙中的纤维振动,导致声能损耗的增大
另外,增强体与基体合金之间的微塑性变形、热膨胀失配甚至界面区域周围的高密度位错也能使声能的损耗增大[26],从而使吸声性能提高
同时,随着纤维含量的提高,穿孔纤维和孔间纤维数量增多,泡沫的曲折度[34]、孔壁粗糙度、比表面积以及孔隙中空气的流动阻力都随之增大,摩擦和粘滞性损耗增大,使吸声性能提高;但是,过高的纤维含量(高于8%)时吸声性能下降
其原因是,纤维含量过高使纤维的团聚加剧[6]、孔隙数量减少(团聚的纤维结合铝液阻塞孔隙)、次孔尺寸增大和孔隙合并
4 结论
(1) 采用渗流铸造法可制备主孔径为0.35 mm、次孔径为0.12 mm的高孔隙率(77%~86%)ZL104合金泡沫和304不锈钢纤维/ZL104合金复合泡沫;泡沫孔结构中的次孔分布在主孔壁上形成了多孔孔壁,复合泡沫孔结构中的纤维以孔壁纤维、孔间纤维和穿孔纤维三种状态存在
(2) 纤维复合提高了复合泡沫的力学性能
孔隙率一定时,复合泡沫的屈服强度随着纤维含量的提高先提高后降低;复合泡沫受力时孔壁纤维和穿孔纤维能降低应力集中,纤维以位移和偏转等方式消耗能量而使其力学性能提高
(3) 纤维复合提高了复合泡沫的吸声性能
在1000~5800 Hz复合泡沫的吸声系数随着孔隙率和纤维含量的提高先提高后降低
根据J-A模型,孔隙率、孔径、曲折因子、声频及材料厚度一定的复合泡沫,纤维复合使其孔壁粗糙度提高和比表面积增大,摩擦和粘滞作用使声波的损耗增大,多孔孔壁结构进一步增大声能损耗从而使其吸声性能提高
参考文献
View Option 原文顺序文献年度倒序文中引用次数倒序被引期刊影响因子
[1]
Bai P, Yang X, Shen X, et al.
Sound absorption performance of the acoustic absorber fabricated by compression and microperforation of the porous metal
[J]. Materials & Design, 2019, 167: 107637
[本文引用: 1]
[2]
Marx J C, Robbins S J, Grady Z A, et al.
Polymer infused composite metal foam as a potential aircraft leading edge material
[J]. Applied Surface Science, 2020, 505: 144114
DOIURL
[3]
Otaru A J, Morvan H P, Kennedy A R.
Modelling and optimisation of sound absorption in replicated microcellular metals
[J]. Scripta Materialia, 2018, 150: 152
DOIURL
[4]
Shen X, Bai P, Chen L, et al.
Development of thin sound absorber by parameter optimization of multilayer compressed porous metal with rear cavity
[J]. Applied Acoustics, 2020, 159: 107071
DOIURL
[5]
Otaru A J, Morvan H P, Kennedy A R.
Numerical modelling of the sound absorption spectra for bottleneck dominated porous metallic structures
[J]. Applied Acoustics, 2019, 151: 164
DOI [本文引用: 1]

Numerical simulations are used to test the ability of several common equivalent fluid models to predict the sound absorption behaviour in porous metals with "bottleneck" type structures. Of these models, Wilson's relaxation model was found to be an excellent and overall best fit for multiple sources of experimental acoustic absorption data. Simulations, incorporating Wilson's model, were used to highlight the relative importance of key geometrical features of bottleneck structures on the normal incidence sound absorption spectrum. Simulations revealed significant improvements in absorption behaviour would be achieved, over a "benchmark" structure from the literature, by maximising the porosity (0.8) and targeting a permeability in the range of 4.0 x 10(-10) m(2). Such a modelling approach should provide a valuable tool in the optimisation of sound absorption performance and structural integrity, to meet application-specific requirements, for a genre of porous materials that offer a unique combination of acoustic absorption and load bearing capability. (C) 2019 Elsevier Ltd.
[6]
Wang Y, Zuo X, Kong D, et al.
A comparative analysis of sound absorption performance of ZL104/aluminum fiber composite foam
[J]. J. Mater. Res., 2019, 34(21): 3717
DOIURL [本文引用: 3]
[7]
Kim S Y, Park S H, Um Y S, et al.
Sound absorption properties of Al foam
[J]. Materials Science Forum, 2005, 468: 486
[本文引用: 1]
[8]
Haward S J, Odell J A, Li Z, et al.
The rheology of polymer solution elastic strands in extensional flow
[J]. Rheol. Acta., 2010, 49(7): 781
DOIURL
[9]
Zhai W, Yu X, Song X, et al.
Microstructure-based experimental and numerical investigations on the sound absorption property of open-cell metallic foams manufactured by a template replication technique
[J]. Materials & Design, 2018, 137: 108
[10]
Liu P S, Qing H B, Hou H L.
Primary investigation on sound absorption performance of highly porous titanium foams
[J]. Materials & Design, 2015, 85(Nov.15) : 275
[11]
Bai P, Shen X, Zhang X, et al.
Influences of compression ratios on sound absorption performance of porous nickel-iron alloy
[J]. Metals, 2018, 8(7): 539
DOIURL

The improvement of sound absorption performance of porous metal is a focus of research in the field of noise reduction. Influences of compression ratios on sound absorption performance of a porous nickel–iron (Ni–Fe) alloy were investigated. The samples were compressed with ratios from 10% to 80% at an interval of 10%. Based on the standing wave method, sound absorption coefficients of compressed samples with different thicknesses were obtained. It could be found that with the same compression ratio, sound absorption performance was improved with the increase of thickness. Based on the modified Johnson–Allard model with a correction factor, the sound absorption coefficient of the porous Ni–Fe with a thickness of 20 mm for different compression ratios was derived, whose aim was to quantificationally analyze influences of the compression ratio. The results indicated that the sample with a compression ratio of 70% exhibited optimal sound absorption performance, and its average sound absorption coefficient reached 88.97% in a frequency range of 1000–6000 Hz. Meanwhile, the section morphologies of compressed samples were investigated by a scanning electron microscope, which studied the sound absorption performance by analyzing structures of the porous Ni–Fe samples with different compression ratios. The obtained achievements will promote the application of the porous Ni–Fe alloy in the field of acoustics.
[12]
Liu P S, Qing H B.
A Spherical-pore foamed titanium alloy with high porosity
[J]. Chinese Journal of Materials Research, 2015, 29(5): 346
[本文引用: 1]
刘培生, 顷淮斌.
一种具有球形孔隙的高孔率泡沫钛合金
[J]. 材料研究学报, 2015, 29(5): 346
[本文引用: 1]
[13]
Jin W, Liu J, Wang Z, et al.
Sound absorption characteristics of aluminum foams treated by plasma electrolytic oxidation
[J]. Materials (Basel), 2015, 8(11): 7511
DOIURL [本文引用: 1]
[14]
Navacerrada M A, Fernández P, Díaz C, et al.
Thermal and acoustic properties of aluminium foams manufactured by the infiltration process
[J]. Applied Acoustics, 2013, 74(4): 496
DOIURL
[15]
Ao Q B, Wang J Z, Tang H P, et al.
Sound absorption characteristics and structure optimization of porous metal fibrous materials
[J]. Rare Metal Materials and Engineering, 2015, 44(11): 2646
DOIURL
[16]
Perrot C, Chevillotte F, Panneton R.
Bottom-up approach for microstructure optimization of sound absorbing materials
[J]. J. Acoust. Soc. Am., 2008, 124(2): 940
DOIPMID

Results from a numerical study examining micro-/macrorelations linking local geometry parameters to sound absorption properties are presented. For a hexagonal structure of solid fibers, the porosity phi, the thermal characteristic length Lambda('), the static viscous permeability k(0), the tortuosity alpha(infinity), the viscous characteristic length Lambda, and the sound absorption coefficient are computed. Numerical solutions of the steady Stokes and electrical equations are employed to provide k(0), alpha(infinity), and Lambda. Hybrid estimates based on direct numerical evaluation of phi, Lambda('), k(0), alpha(infinity), Lambda, and the analytical model derived by Johnson, Allard, and Champoux are used to relate varying (i) throat size, (ii) pore size, and (iii) fibers' cross-section shapes to the sound absorption spectrum. The result of this paper tends to demonstrate the important effect of throat size in the sound absorption level, cell size in the sound absorption frequency selectivity, and fibers' cross-section shape in the porous material weight reduction. In a hexagonal porous structure with solid fibers, the sound absorption level will tend to be maximized with a 48+/-10 microm throat size corresponding to an intermediate resistivity, a 13+/-8 microm fiber radius associated with relatively small interfiber distances, and convex triangular cross-section shape fibers allowing weight reduction.
[17]
Yang X H, Ren S W, Wang W B, et al.
A simplistic unit cell model for sound absorption of cellular foams with fully/semi-open cells
[J]. Composites Science and Technology, 2015, 118:276
DOIURL
[18]
Guan D, Wu J H, Jing L.
A statistical method for predicting sound absorbing property of porous metal materials by using quartet structure generation set
[J]. Journal of Alloys and Compounds, 2015, 626: 29
DOIURL
[19]
Soni B, Biswas S.
Effects of cell parameters at low strain rates on the mechanical properties of metallic foams of Al and 7075-T6 alloy processed by pressurized infiltration casting method
[J]. J. Mater. Res., 2018, 33(20): 3418
DOIURL [本文引用: 1]
[20]
Yu X, Lu Z, Zhai W.
Enhancing the flow resistance and sound absorption of open-cell metallic foams by creating partially-open windows
[J]. Acta Materialia, 2021, 206: 116666
DOIURL [本文引用: 1]
[21]
Wu J, Cheng H F, Huang X M, et al.
Effects of modification and heat treatment on energyabsorption capacities of aluminum alloy foams
[J]. Special Casting & Nonferrous Alloys, 2011, 31(4): 373
[本文引用: 2]
吴 进, 程和法, 黄笑梅 等.
变质处理及热处理对泡沫铝合金压缩吸能性的影响
[J]. 特种铸造及有色合金, 2011, 31(4): 373
[本文引用: 2]
[22]
Wang H, Zhou X Y, Long B, et al.
Sound absorption properties of open-cells aluminum foams prepared by infiltration casting methods
[J]. The Chinese Journal of Nonferrous Metals, 2013, 23(4): 1034
[本文引用: 1]
王 辉, 周向阳, 龙 波 等.
渗流铸造法制备的开孔泡沫铝的声学性能
[J]. 中国
有色金属学报, 2013, 23(4): 1034
[本文引用: 1]
[23]
Liu M, Cheng H F, Huang X M, et al.
Preparation of low density open-cell aluminum foam by soluble plaster mould
[J]. Special Casting & Nonferrous Alloys, 2010, 30(5): 462
[本文引用: 1]
刘 铭, 程和法, 黄笑梅 等.
用可溶石膏型预制块制备低密度开孔泡沫铝
[J]. 特种铸造及有色合金, 2010, 30(5): 462
[本文引用: 1]
[24]
Alt?nk?k N.
Investigation of mechanical and machinability properties of Al2O3/SiCp reinforced Al-based composite fabricated by stir cast technique
[J]. J. Porous. Mater., 2015, 22(6): 1643
DOIURL [本文引用: 2]
[25]
Guo C, Zou T, Shi C, et al.
Compressive properties and energy absorption of aluminum composite foams reinforced by in-situ generated MgAl2O4 whiskers
[J]. Materials Science and Engineering: A, 2015, 645: 1
DOIURL [本文引用: 1]
[26]
Wu J, Li C, Wang D, et al.
Damping and sound absorption properties of particle reinforced Al matrix composite foams
[J]. Composites Science and Technology, 2003, 63(3-4): 569
DOIURL [本文引用: 1]
[27]
Zhang J, Jie J, Lu Y, et al.
Fabrication of carbon fibers reinforced Al-matrix composites in pulsed magnetic field
[J]. J. Mater. Res. Technol., 2021, 11: 197
DOIURL [本文引用: 1]
[28]
Mu Y, Yao G, Cao Z, et al.
Strain-rate effects on the compressive response of closed-cell copper-coated carbon fiber/aluminum composite foam
[J]. Scripta Materialia, 2011, 64(1): 61
DOIURL [本文引用: 2]
[29]
Huang Y, Ouyang Q, Zhang D, et al.
Carbon materials reinforced aluminum composites: a review
[J]. Acta Metall. Sin. (Engl. Lett.), 2014, 27(5): 775
DOIURL
[30]
Singh B B, Balasubramanian M.
Processing and properties of copper-coated carbon fibre reinforced aluminium alloy composites
[J]. Journal of Materials Processing Technology, 2009, 209(4): 2104
DOIURL
[31]
Pan L W, Rao D W, Yang C, et al.
Research progress on preparation methods and energy absorption properties of hollow particles/metal matrix syntactic foams
[J]. Acta Materiae Compositae Sinica, 2020, 37(6): 1370
[本文引用: 1]
潘利文, 饶德旺, 杨 超 等.
空心微珠/金属基复合泡沫制备方法与吸能性能的研究进展
[J]. 复合材料学报, 2020, 37(6): 1370
[本文引用: 1]
[32]
Lee S, Kim G, Kim H, et al.
Impact resistance, flexural and tensile properties of amorphous metallic fiber-reinforced cementitious composites according to fiber length
[J]. Constr. Build. Mater., 2021, 271: 121872
DOIURL [本文引用: 1]
[33]
Allard J F, Atalla N. Propagation of Sound in Porous Media: Modelling sound absorbing materials 2nd Ed. [M].
Hoboken:
A John Wiley and Sons, 2009: 45
[本文引用: 1]
[34]
Kalauni K, Pawar S J.
A review on the taxonomy, factors associated with sound absorption and theoretical modeling of porous sound absorbing materials
[J]. J. Porous Mater., 2019, 26(6): 1795
DOI [本文引用: 1]
Sound absorption performance of the acoustic absorber fabricated by compression and microperforation of the porous metal
1
2019
声明:
“304不锈钢纤维/ZL104铝合金复合泡沫的孔结构、力学、吸声性能及其机理” 该技术专利(论文)所有权利归属于技术(论文)所有人。仅供学习研究,如用于商业用途,请联系该技术所有人。
我是此专利(论文)的发明人(作者)